Neutron Camera
As the name implies a neutron camera allows detection and recording of the
2-dimensional neutron distribution. However, the neutron is not directly
detected as light is in a standard camera. Here a secondary converter called a
scintillator converts neutrons to light and this light is then recorded with a
camera or digitizing device capable of recording light.
Neutron To Light/Electron Conversion:
The Scintillator (neutron to light conversion)
There are numerous materials that work as a neutron to light converter and all
of them require a phosphor material to transform the energy of charged
particles, from a nuclear reaction between the neutron and an element embedded
in the phosphor (examples are Gd or 6Li), into light. Common
examples of scintillator material include GdOS and 6LiZnS. Although
the neutron is a very point like particle the light event is not and this fact
determines the ultimate spatial resolution that can be obtained with
scintillator materials. Although the range of the charged particles from the
nuclear reaction can be quite short (10 µm to 20 µm), the light from
the scintillation event can bloom to 100 µm to 200 µm. This blooming
length scale is directly proportional to the thickness of the scintillator
screen (typically 100 µm to 200 µm thick). The only way the range of
this blooming can be reduced is to reduce the screen thickness.
However, lowering the screen thickness also lowers the amount of absorbing
converter material that the neutron travels through and therefore the neutron
detection efficiency is proportionately reduced. A more advanced converter
material could in principle localize the event on the same scale as the range
of the charged particles (10 µm to 20 µm), which would be the
ultimate limiting length scale for a neutron imaging device.
Advanced converters (neutron to electron conversion)
Currently under development advanced glass fiber technologies can convert
neutrons to electrons that can be detected with an electron centroiding d
etector. These detectors are able to confine the neutron detection event and
avoid the issue of blooming and thereby achieve resolution of order
(10 µm to 20 µm).
The Digitizer
To capture the image of the light created by the scintillator there are two
types of imagers. The first is a charge coupled device (CCD) type camera with a
normal photographic quality focusing lens. The second is an amorphous silicon
detector designed for harsh radiation environments. Since most electronics
devices are sensitive to radiation damage it is necessary to protect the device
from the neutron beam. The CCD camera can be easily protected by mounting it
outside the beam at a 90 degree angle. The camera then focuses on the
light image formed on the scintillator screen via a photographic lens. Due to
the distance the camera is from the screen much of the light from the
scintillator is lost, which requires long exposure times for the CCD to acquire
an image (several seconds). To run a much higher speeds (30 frames per
second) another system is employed that allows the sensor part to be mounted
directly in the neutron beam. This is an amorphous silicon detector. This type
of sensor is resistant to radiation damage. The electronics used to read the
sensor are not radiation hard and are therefore designed by the manufacturer to
be outside of the beam.
CCD Neutron Camera |
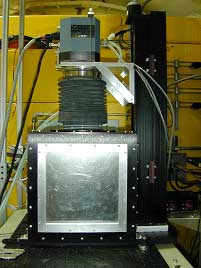
Photo |
Schematic
- Point source of neutrons
- Neutron to light converter
- First surface mirror (45 degree)
- Light tight box
- Standard camera lens
- Peltier cooled CCD chip
- Computer
|
Varian Flat Panel
|
Photograph |
- Amorphous silicon
- Radiation hard
- High frame rate (30 fps)
- 127 µm spatial resolution
- No-optics - scintillator directly couples to the sensor to optimize light
input efficiency
|
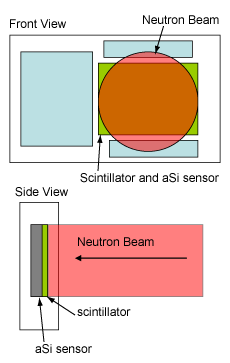
Schematic
|
|
aSi movie: [14 MB Get a
free Media Player]
Shown here is a neutron radiography movie of helium bubbling through water
taken at 30 frames per second demonstrating the dynamic capabilities of
this detector.
|
|
|
|
Peltier Effect
The Peltier Effect was discovered in 1834 by a French watchmaker and physicist
by the name of Jean Charles Athanase Peltier (1785-1845). The Peltier Effect
follows from a related effect discovered in 1821 by Thomas Johann Seebeck who
showed that if heat is applied to a circuit at the junction of two different
conductors a current will be generated. In 1834 Peltier demonstrated the
converse effect (the Peltier effect). He found that when a current is passed
through a circuit of two dissimilar conductors there will be a rise or fall in
temperature at the junction depending on the direction of the current flow. One
junction between the two materials becomes warm while the other cool. This
electrical pumping of heat from one side of a device to the other is also known
as thermoelectric cooling and is capable of cooling CCD chips to temperatures
as low as -60 °C.
Inquiries or comments:
David
Jacobson
Online: July 2004 - Last update: May 2006
|